Chondrogenic differentiation followed IGFBP3 loss in human endometrial mesenchymal stem cells
Biochemical and Biophysical Research Communications
j o u r n a l h o m e p a g e : w w w . e l s e v i e r . c o m/ l o c a t e / y b b r c
Roman E. Ushakov, Elena V. Skvortsova, Mikhail A. Vitte, Irina O. Vassilieva b, Alla N. Shatrova, Anastasiya V. Kotova, Vladimir M. Kenis, Elena B. Burova,Institute of Cytology RAS, Tikhoretsky Ave 4, St. Petersburg, 194064, Russia
City Clinical Hospital N 31, Dynamo ave 3, St. Petersburg, 197110, Russia
H. Turner National Medical Research Center for Children’s Orthopedics and Trauma Surgery, Parkovaya 64-68, Pushkin, St. Petersburg, 196603, Russia
Abstract
Insulin-like growth factor binding protein 3 (IGFBP3) is a multifunctional protein, able either to stimulate the cell growth or to promote apoptosis. In particular, IGFBP3 plays significant role in propagation of stress-induced senescence in human endometrium-derived mesenchymal stem cells (MESCs) (Vassilieva et al., 2020). We undertook CRISPR/Cas9-mediated IGFBP3 knockout in an effort to decelerate stress-induced senescence in MESCs, but, unexpectedly, IGFBP3-knockout MESCs culture acquired chondrocyte-like features, such as cell condensation and aggregation. We revealed that IGFBP3-knockout MESCs completely lost CD73 and CD90 MESCs positive surface markers, and significantly decreased expression of CD105 and CD146 MESCs positive surface markers. In addition, we found IGFBP3-knockout MESCs aggregates positively stained for Alcian Blue. We also detected expression of collagen type II in IGFBP3-knockout MESCs. The obtained results indicate that MESCs lost stemness after IGFBP3-knockout and underwent differentiation toward chondrogenic lineage. Our findings can enlighten IGFBP3 role in regulation of MESCs chondrogenesis.
1. Introduction
Mesenchymal stem cells (MSCs) are postnatal multipotent stem cells which are able to differentiate into osteoblasts, chondrocytes and adipocytes. MSCs, settling so called niches throughout the body, support a tissue repair due to self-renewal capacity and modulate immune functions in vivo. MSCs show promise for regenerative medicine because of their secretory phenotype, immunomodulating properties and ability to differentiation to-ward multiple cell types from all three embryonic germ layers. MSCs-based therapy involves isolation of MSCs from donor’s tissue and expansion in vitro followed by MSCs transplantation to the patient. Depending on therapy purposes, MSCs can be tenta-tively preconditioned or genetically modified. A wide range of adult tissues and secretions including bone marrow, dental pulp, adipose tissue, mother’s milk was reported as MSCs source. Our research focuses on properties of human endometrium-derived mesenchymal stem cells (MESCs) obtained from men-strual blood.
Earlier, we have reported that MESCs undergo the premature senescence under oxidative stress. Like the other MSCs, cell senescence affects MESCs, impairing their biological functions and leading to significant changes in secretome composition. Senescence associated secretory phenotype (SASP) factors promote senescence of neighboring cells. In this occasion, MESCs therapeutic action is possibly denied. In the foregoing article, we have investigated the role of insulin-like growth factor (IGF) binding protein 3 (IGFBP3) in the paracrine senescence propagation in MESCs culture. IGFBP3, being a SASP factor, is upregulated in senescent cells, thereby its involvement in senescence spread might be suggested.
IGFBP3, protein of our interest, is a member of IGFBPs super-family. IGFBPs carry out different functions, depending on func-tional motifs collection, such as binding sites for heparin, cell surface proteoglycans, different components of extracellular ma-trix, sites of post-translational modifications, proteolytic cleavage sites, etc. Furthermore, some IGFBPs including IGFBP3 contain nu-clear localization sequences (NLS), so that they could be trans-ported into the nucleus in certain cell types.
Being secreted into the extracellular environment, the IGFBPs inhibit or stimulate cell growth by altering an accessibility of extracellular IGFs to their receptors on cell surface. The IGFBPs are bound with IGFs either in a binary complex or a ternary complex contained IGF, IGFBP3 (rarely IGFBP5), and a glycoprotein called acid labile subunit (ALS) what prevents complex biodegra-dation. Also, the IGFBPs are capable to affect cell proliferation, migration, differentiation, angiogenesis, and apoptosis in IGF/IGF-1R independent manner. IGFBPs action covers extracel-lular as well as intracellular space. In this way, the row of potential IGFBP3 interactants is quite big. The IGFBP3 function to an important extent depends on cellular context. IGFBP3 could facili-tate DNA repair and stimulate the cell growth as well as promote apoptosis if genome integrity is compromised.
Clustered regularly interspaced short palindromic repeats (CRISPR) and their CRISPR-associated (Cas) proteins are an essential part of bacteria and archaea adaptive immune system. Cas proteins directed by specific guide RNA cut DNA, creating double-strand breaks (DSBs). Non-homologous end joining (NHEJ) DNA repair pathway typically results in small insertions and/or deletions (INDELs) creation, leading to a frameshift mutation as well as shutting the targeted gene off.
In our recent study, involvement of IGFBP3 secreted from old cells in paracrine senescence of neighboring cells in MESCs culture was clearly demonstrated. To confirm this phenomenon, it was interesting to evaluate paracrine activity of IGFBP3-knockout MESCs. Using CRISPR/Cas9 technology to produce such cells has been not described yet. Surprisingly, CRISPR/Cas9-mediated IGFBP3 gene knockout resulted in dramatic changes of MESCs phenotype. Additionally, according to preliminary data knockout cells acquired greater sensitivity to oxidative stress and at the same time lost an ability to senesce. Taken together, these findings prompted us to investigate peculiar properties of IGFBP3-knockout MESCs in detail. So, the current research is aimed to elucidate a fate of MESCs subjected to genome editing.
2. Materials and methods
2.1. MESCs culture
Human endometrium-derived mesenchymal stem cells (MESCs, line 2804) obtained from menstrual blood of healthy donor and characterized previously were cultured as previously described. Cells were trypsinized and plated at following densities: 120 103 per cm2 for transfection, 15 103 cm2 for protein extraction and 10 103 per cm2 on glass coverslips for immunocytochemistry.
2.2. Isolation and culture of human primary chondrocytes
All procedures involving human beings complied with the ethical standards of the institutional and/or national research ethics committee and the 1964 Helsinki Declaration and its sub-sequent changes or comparable ethical standards. An informed written consent was obtained from each of the participants enrolled in the study (or his/her parents for underage children). Specimens (2e3 mm2) were collected from donors without genetic diseases during surgical treatment of knee joints. A cartilage frag-ment was washed with PBS and minced into small pieces by sur-gical scalpel before 3-h digestion with 0.1 mg/ml Collagenase II Type (Thermo Fisher Scientific, USA) in PBS at 37 C in shaker-incubator. The cartilage fragments were washed 3 times with PBS by centrifugation and added to supernatants, then the mix was centrifuged for 7 min at 400 g. The pellet was resuspended in a low glucose DMEM (DMEM LG GlutaMAX, Gibco, USA) supplemented with 10% FBS, 100 U/ml penicillin, and 100 mg/ml streptomycin and plated to 25 cm2 flask. After 3e5 days the culture medium was changed to Chondrocyte Basal Medium (PromoCell, Germany) with 10% Supplement (PromoCell, Germany). Medium was refreshed every 3 days. The primary culture (Passage 0) reached confluency after 5e10 days, then was harvested by trypsinization and frozen in CryoStor CS10 (STEMCELL Technologies, Canada). Thawed chon-drocytes were cultivated in Chondrocyte Basal Medium at 37 C in a humidified 5% CO2 atmosphere, and then were used for RNA isolation.
2.3. IGFBP3 gene knockout
MESCs were seeded on 12-well plate 18 h before experiment. Cells were co-transfected with 2.5 mg/ml cocktail of CRISPR/Cas9 plasmids, carrying IGFBP3-specific sgRNA sequences (IGFBP3 CRISPR/Cas9 KO Plasmid (h2), Santa Cruz Biotechnology, USA), and with 2.5 mg/ml plasmid conferring resistance to puromycin (HDR CRISPR/Cas9 Plasmid, Santa Cruz Biotechnology, USA). Transfection was performed with use of 5 ml/ml of Lipofectamine Stem Trans-fection Reagent (Thermo Fisher Scientific, USA) according to man-ufacturer’s instructions. To obtain stable cell line, 5 days after transfection cells were selected for 5 days with 2 mg/ml puromycin. After selection, cells were cultivated in growth medium supple-mented with 20% FBS.
2.4. Immunoblotting
Cell lysis, protein sample preparation, SDS-PAGE electrophoresis and western blotting including ECL detection were carried out as described previously. Primary antibodies against IGFBP3 (R&D Systems, USA) and GAPDH (Cell Signaling Technology, USA) were utilized.
2.5. Immunophenotyping
Analysis of cell surface markers was conducted as described previously. Cell samples stained with PE and FITC conjugated antibodies to positive MESCs markers CD73, CD90, CD105, CD140, CD146 and to negative MESCs markers CD34, CD45, HLA-DR were analyzed using CytoFlex S flow cytometer (Beckman Coulter, Brea, CA, USA). Gathered data were processed with CytExpert v 2.0 software.
2.6. Immunocytochemistry
Immunofluorescence staining was performed as described previously. Primary antibodies to vimentin, nestin, alpha-fetoprotein, E-cadherin, cytokeratin 5, cytokeratin 14, cytokeratin 15, neurofilament light chain (NF-L), beta-tubulin III and collagen type II were used. Goat anti-mouse and goat anti-rabbit secondary antibodies conjugated to Alexa488 and Alexa568 were utilized. Fluorescent images were taken by means of Zeiss Axiovert 200 Minverted fluorescence microscope (Zeiss, Gottingen,€ Germany).
2.7. Chondrogenic and osteogenic differentiation
In order to induce osteogenic and chondrogenic differentiation of MESCs, StemPro Osteogenesis Differentiation Kit and StemPro Chondrogenesis Differentiation Kit (Thermo Fisher Scientific, USA) were used according to manufacturer’s instructions.
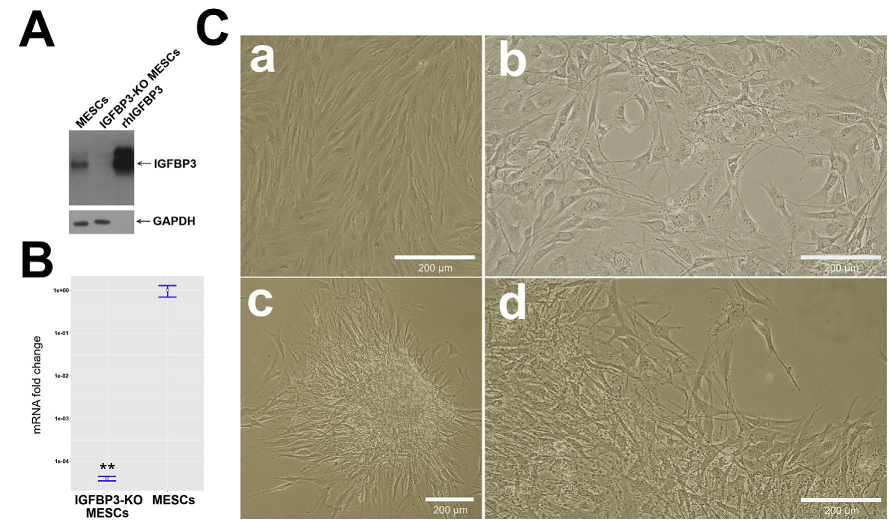
2.8. Alizarin Red and Alcian Blue staining
To reveal calcium deposits, IGFBP3-KO MESCs seeded on plate were washed with PBS, fixed with ethanol for 40 min, washed two times with distilled water, stained with 2% Alizarin Red solution adjusted to pH 4.3, then washed with distilled water again. MESCs cultivated for 21 day in osteogenic differentiation medium were used as positive control of Alizarin Red staining. In order to detect glycosaminoglycans, IGFBP3-KO MESCs were washed with PBS, fixed with 10% neutral buffered formalin, then washed with distilled water, stained for 30 min with 1% Alcian Blue solution prepared in 3% acetic acid, washed two times with distilled water. MESCs cultivated for 21 day in chondrogenic differentiation me-dium were used as positive control of Alcian Blue staining.
2.9. Analysis of gene expression
RNA extraction from cell cultures and quantitative RT-PCR were performed as described previously [10]. The primer sequences used for IGFBP3: 50-TCACCTGAAGTTCCTCAATGT-3ʹ (forward) and 50-ACTTATCCACACACCAGCAGA-3ʹ (reverse); for COL2A1: 50-GGCAA-TAGCAGGTTCACGTACA-3ʹ (forward) and 50-CGATAA-CAGTCTTGCCCCACTT-3ʹ (reverse); for ACAN: 50-GCTACGACGCCATCTGCTAC-3ʹ (forward) and 50-GTCAGGCCAGGT-CACTGTC-3ʹ (reverse); for COL1A1: 50-GACCTAAAGGTGCTGCTG-GAG-3ʹ (forward) and 50-CTTGTTCACCTCTCTCGCCA-3ʹ (reverse), for reference gene GAPDH: 50-GAAATCCCATCACCATCTTCCAGG-3ʹ (forward) and 50-GAGCCCCAGCCTTCTCCATG-3ʹ (reverse). All primers were obtained from Syntol (Russia).
2.10. Statistical analysis
Numerical data are presented as mean values of at least three independent experiments with standard deviations if other is not indicated. Unpaired two-samples Student’s t-test and one-way
ANOVA with post-hoc Tukey test were used for groups compari-son. Significance level was set on p < 0,05.
3. Results
3.1. Knockout cell culture
IGFBP3 knockout was confirmed at both protein (Fig. 1A) and mRNA levels (Fig. 1B). About 6 weeks after IGFBP3 knockout sig-nificant changes of cell morphology and growth pattern were spotted. Unlike spindle-shaped intact MESCs constituting fingerprint-like monolayer, knockout cells demonstrate more va-riety in shape, tend to aggregate and to form colonies (Fig. 1C). Interestingly, colony-forming ability of knockout cells depends on seeding density: at low density cells take up culture surface equally and by reaching certain density threshold cells start creeping together to edge of culture dish and forming colonies.
3.2. Loss of MESCs multipotency markers
We analyzed expression of some MESCs characteristic surface markers on knockout cells and revealed complete loss of typical for MESCs CD73 and CD90 positive markers and remarkable decrease (up to 2 times) of CD105 and CD146 in comparison to intact MESCs (Fig. 2A and B), while negative MESCs markers CD34, CD45 and HLA-DR were not affected. Besides that, nestin and neurofilament light chain (NF-L) presenting in intact MESCs described previously as predisposed to neurogenic differentiation vanished in knockout cells (Fig. 3A). Summarizing, MESCs seemed to lose their stemness after IGFBP3 knockout.
3.3. Cell type recognition
Taking into account the knockout cell culture metamorphosis and loss of stemness, we supposed that knockout MESCs
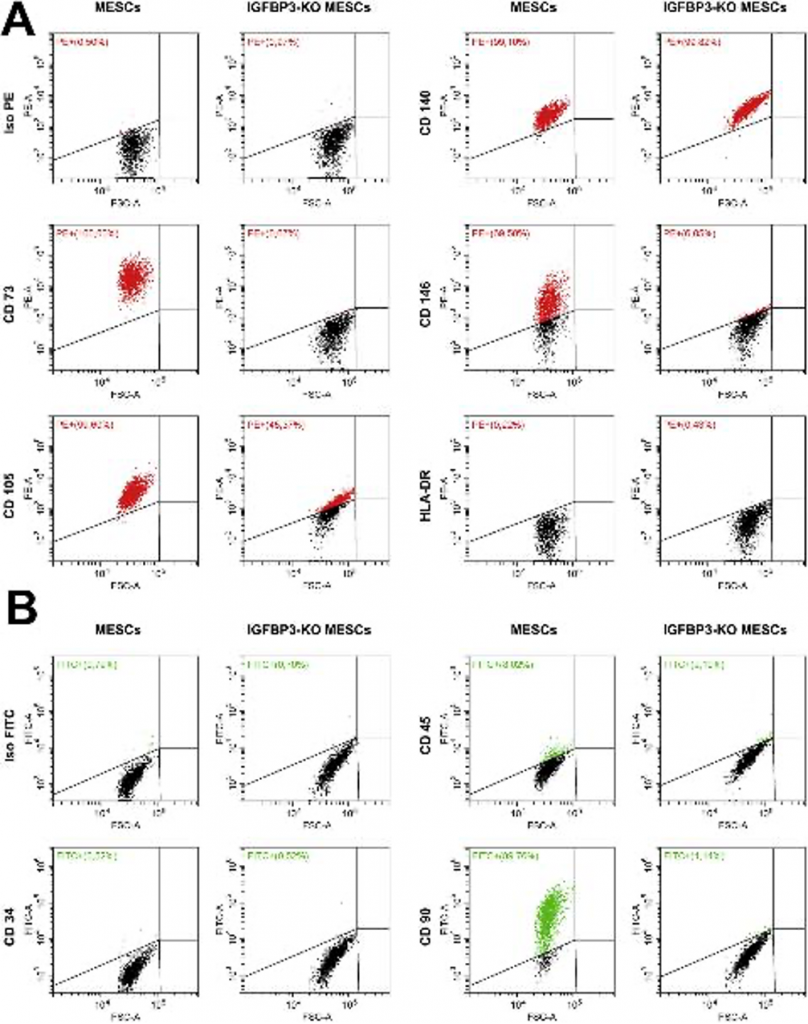
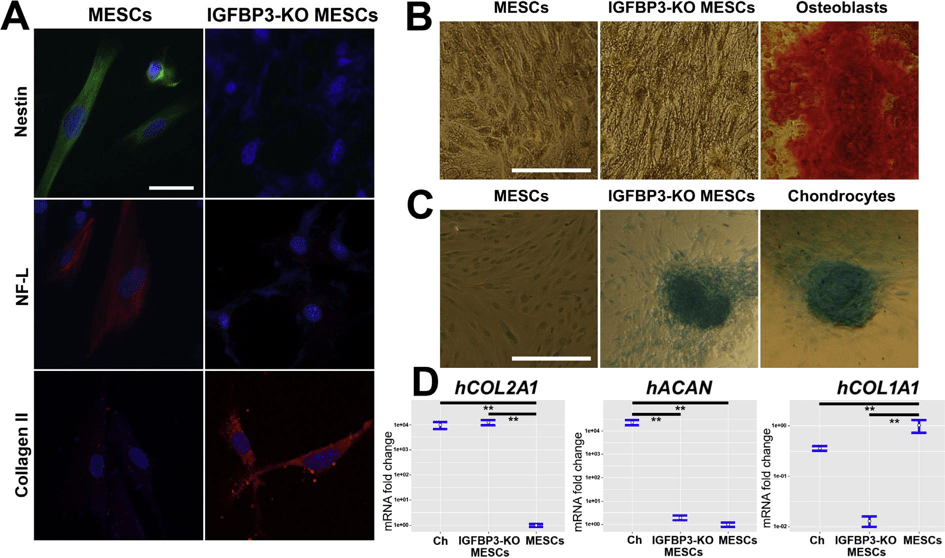
underwent differentiation; therefore, we attempted to identify cell type. Assumptions about mesenchymal-epithelial transition, neurogenic differentiation and transdifferentiation to endoderm lineage were sequentially denied because of negative staining of knockout cells with antibodies against E-cadherin, cytokeratin 5, cytokeratin 14, cytokeratin 15, beta-tubulin III and alpha-fetoprotein (data not shown).
Next, knockout cell culture staining with Alizarin Red did not reveal any calcium deposits (Fig. 3B). Eventually, Alcian Blue staining of knockout cell aggregates was positive (Fig. 3C) exhibit-ing presence of glycosaminoglycans that are characteristic for chondrocytes. We tested expression of cartilage-specific collagen type II to verify whether knockout cells differentiated toward chondrogenic lineage. We found that collagen type II mRNA level in knockout cells is significantly higher than in MESCs and it is com-parable to collagen type II mRNA level of primary human chon-drocytes (Fig. 3D). Presence of collagen type II in knockout cells was also confirmed with immunofluorescent staining (Fig. 3A). In addition, knockout MESCs demonstrated significant decrease in mRNA collagen type I expression (Fig. 3D). We checked also expression level of cartilage-specific proteoglycan aggrecan in knockout MESCs, but it is as low as in native MESCs and it cannot be matched with aggrecan level in primary chondrocytes (Fig. 3D).
4. Discussion
Regulation of MSCs differentiation is one of the key aspects of MSCs biology. Many in vitro studies shed light on complex nature of this phenomenon which is affected by broad spectrum of genetic, physical and biochemical influences. Above all, MSCs commitment to one or another lineage requires activation of spe-cific transcription factors. This process is mediated by various extracellular stimuli (such as growth factors, hormones, cytokines) employing multiple signaling pathways. These stimuli include insulin-like growth factors acting via their own receptors and insulin receptor. IGFBPs can modulate IGFs ef-fects by regulation of availability of IGFs ligands for interacting with receptors. However, IGFBPs demonstrate numerous IGF-independent actions as well. IGFBP3, center of our interest, is reported to modulate differentiation of myoblasts, odonto-blasts, osteoblasts, preadipocytes, it promotes also fibroblast-to-myofibroblast transition in diseased prostatic tissue. IGFBP3 knockdown in zebrafish embryos reduced differenti-ation of pharyngeal cartilage. IGFBP3 effects on differentiation are controversial in various in vitro models, and depending on cell type IGFBP3 can both support and inhibit differentiation. To our knowledge, there are still no published data on IGFBP3 role in differentiation of endometrium-derived MSCs.
Several weeks after MESCs transfection with CRISPR/Cas9 plasmid that led to successful IGFBP3 knockout we observed un-expected changes of cell culture. Considering loss of multipotency markers, cell aggregation, positive staining of aggregates with Alcian Blue and expression of collagen type II, we suppose that IGFBP3-knockout MESCs differentiated toward chondrogenic line-age. However, like intact MESCs, knockout MESCs do not express aggrecan.
Remarkably, IGFBP3-knockout MESCs demonstrated chondrocyte-like phenotype, although they were cultured in standard conditions without any chondroinductive supplements. Possible explanation of observed phenomenon could be following: since IGFBP3 neutralizes IGF-1 by direct binding, IGFBP3-lacking MESCs might be more sensitive to IGF-1, which is known as fac-tor promoting chondrogenesis in MSCs, and in this way IGF-1 presenting in complete growth medium could contribute to chondrogenic differentiation.
IGFBP3 protein was shown to be a negative regulator of prolif-eration and differentiation of mesenchymal chondroprogenitors in works of Spagnoli and colleagues. They studied unique cell population designated RCJ3.1C5.18 obtained by Grigoriadis and coworkers by limiting dilution from fetal rat calvaria cells. These cells differentiate in vitro into three-dimensional cartilage nodules synthesizing collagen type II and glycosaminoglycans in standard medium within 14 days. Interestingly, RCJ3.1C5.18 express neither IGFs nor IGFBP3, for this reason this cell line is considered as ideal model for study of IGF-independent IGFBP3 effects without interference from endogenous peptides. It has been shown that IGFBP3 specifically reduces proliferation of RCJ3.1C5.18 in dose-dependent manner and this effect is IGF-independent during early stages of differentiation. IGFBP3 exerts IGF-independent antiproliferative action through upregula-tion and activation of STAT-1 leading to apoptosis. IGFBP3 decelerates differentiation of RCJ3.1C5.18 and inhibits chon-droinductive action of TGF-b, factor which is commonly used for in vitro chondrogenic differentiation of MSCs. Canonical TGF-b signaling commits MSCs to chondrogenic lineage via Smad pathway, however, negative cross-talk between IGFBP3 and TGF-b in RCJ3.1C5.18 is implemented through MAPK/ERK pathway. It has been hypothesized that IGFBP3 modulates chondrogenesis by controlling number of chondroprogenitors committed to undergo differentiation.
Besides that, IGFBP3 is known to be upregulated in cartilage disorders such as rheumatoid arthritis and osteoarthritis. IGFBP3 is increased in serum and synovial fluid of patients with rheumatoid arthritis. Damaged regions of osteoarthritic cartilage demonstrate high expression level of IGFBP3 in comparison to intact regions from the same joints. IGFBP3 was found in chondrocyte nuclei of cartilage samples taken from patients with osteoarthritis and it was suggested that IGFBP3 regulates gene expression. Furthermore, IGFBP3 is suspected of osteoarthritis triggering by inducing apoptosis in rat chondrocytes independently from IGF-1. On the other hand, expression of IGFBP3 is significantly downregulated in putative chondroprogenitor cells compared with MSCs. IGFBP3 knockdown in ATDC5 chon-drogenesis model system substantially decreased markers of chondrocyte hypertrophy, a characteristic feature of osteoarthritis. Curiously, cartilaginous tumors such as enchondromas and chondrosarcomas demonstrate low levels of IGFBP3 expression compared with normal growth plates.
In conclusion, we found MESCs differentiated toward chondro-genic lineage after IGFBP3 knockout. According to literature, IGFBP3 appears to be a specific factor constraining chondrogenic differ-entiation of mesenchymal progenitors in vitro, it impairs also cartilage metabolism in vivo. It may be assumed that MESCs lacking IGFBP3 are predisposed to chondrogenic differentiation. Although exact mechanisms underlying this phenomenon are not elucidated yet, we suppose that IGFBP3 may play a role in maintaining of MESCs multipotency. Thus, our work may contribute to under-standing of IGFBP3 involvement in regulation of chondrogenesis.
Declaration of competing interest
The authors declare no conflicts of interest.
Acknowledgements
This study was supported by the Russian Foundation for Basic Research Grant (#19-04-00598).
References
[1] E.M. Horwitz, K. Le Blanc, M. Dominici, I. Mueller, I. Slaper-Cortenbach, F.C. Marini, R.J. Deans, D.S. Krause, A. Keating, Clarification of the nomencla-ture for MSC: the international society for cellular therapy position statement, Cytotherapy (2005), https://doi.org/10.1080/14653240500319234.
[2] P. Saeedi, R. Halabian, A.A. Imani Fooladi, A revealing review of mesenchymal stem cells therapy, clinical perspectives and Modification strategies, Stem Cell Investig. (2019), https://doi.org/10.21037/sci.2019.08.11.
[3] R. Hass, C. Kasper, S. Bohm,€ R. Jacobs, Different populations and sources of human mesenchymal stem cells (MSC): a comparison of adult and neonatal tissue-derived MSC, Cell Commun. Signal. (2011), https://doi.org/10.1186/ 1478-811X-9-12.
[4] A. Andrzejewska, B. Lukomska, M. Janowski, Concise review: mesenchymal stem cells: from roots to boost, Stem Cell. (2019), https://doi.org/10.1002/ stem.3016.
[5] V.I. Zemelko, T.M. Grinchuk, A.P. Domnina, I.V. Artzibasheva, V.V. Zenin, A.A. Kirsanov, N.K. Bichevaia, V.S. Korsak, N.N. Nikolsky, Multipotent mesen-chymal stem cells of desquamated endometrium: isolation, characterization, and application as a feeder layer for maintenance of human embryonic stem cells, Cell Tissue Biol (2012), https://doi.org/10.1134/S1990519X12010129.
[6] E. Burova, A. Borodkina, A. Shatrova, N. Nikolsky, Sublethal oxidative stress induces the premature senescence of human mesenchymal stem cells derived from endometrium, Oxid. Med. Cell. Longev. (2013), https://doi.org/10.1155/ 2013/474931.
[7] V. Severino, N. Alessio, A. Farina, A. Sandomenico, M. Cipollaro, G. Peluso, U. Galderisi, A. Chambery, Insulin-like growth factor binding proteins 4 and 7 released by senescent cells promote premature senescence in mesenchymal stem cells, Cell Death Dis. (2013), https://doi.org/10.1038/cddis.2013.445.
[8] S. Ozcan, N. Alessio, M.B. Acar, E. Mert, F. Omerli, G. Peluso, U. Galderisi, Un-biased analysis of senescence associated secretory phenotype (SASP) to identify common components following different genotoxic stresses, Aging (2016), https://doi.org/10.18632/aging.100971. Albany. NY.
[9] H. Kupcova Skalnikova, Proteomic techniques for characterisation of mesen-chymal stem cell secretome, Biochimie (2013), https://doi.org/10.1016/ j.biochi.2013.07.015.
[10] I. Vassilieva, V. Kosheverova, M. Vitte, R. Kamentseva, A. Shatrova, N. Tsupkina, E. Skvortsova, A. Borodkina, E. Tolkunova, N. Nikolsky, E. Burova, Paracrine Senescence of Human Endometrial Mesenchymal Stem Cells: a Role for the Insulin-like Growth Factor Binding Protein 3, Aging, 2020, https:// doi.org/10.18632/aging.102737. Albany. NY.
[11] R.C. Baxter, Insulin-like growth factor binding protein-3 (IGFBP-3): novel li-gands mediate unexpected functions, J. Cell Commun. Signal. (2013), https:// doi.org/10.1007/s12079-013-0203-9.
[12] R.C. Baxter, Insulin-like growth factor binding proteins in the human circu-lation: a review, Horm. Res. Paediatr. (1994), https://doi.org/10.1159/ 000184186.
[13] J.I. Jones, D.R. Clemmons, Insulin-like growth factors and their binding pro-teins: biological actions, Endocr. Rev. (1995), https://doi.org/10.1210/edrv-16-1-3.
[14] S.S. Huang, T.-Y. Ling, W.-F. Tseng, Y.-H. Huang, F.-M. Tang, S.M. Leal, J.S. Huang, Cellular growth inhibition by IGFBP-3 and TGF-b 1 requires LRP-1, FASEB J. (2003), https://doi.org/10.1096/fj.03-0256com.
[15] B. Liu, H.Y. Lee, S.A. Weinzimer, D.R. Powell, J.L. Clifford, J.M. Kurie, P. Cohen, Direct functional interactions between insulin-like growth factor-binding protein-3 and retinoid X receptor-a regulate transcriptional signaling and apoptosis, J. Biol. Chem. (2000), https://doi.org/10.1074/jbc.M002547200.
[16] R. Sorek, C.M. Lawrence, B. Wiedenheft, CRISPR-mediated adaptive immune systems in bacteria and archaea, Annu. Rev. Biochem. (2013), https://doi.org/ 10.1146/annurev-biochem-072911-172315.
[17] R. Barrangou, The roles of CRISPR-Cas systems in adaptive immunity and beyond, Curr. Opin. Immunol. (2015), https://doi.org/10.1016/ j.coi.2014.12.008.
[18] M.C. Negritto, Repairing double-strand DNA breaks, Nat. Educ. (2010).
[19] D. Cook, P. Genever, Regulation of mesenchymal stem cell differentiation, Adv.
Exp. Med. Biol. (2013), https://doi.org/10.1007/978-94-7-6621-1_12.
[20] A. Augello, C. De Bari, The regulation of differentiation in mesenchymal stem cells, Hum. Gene Ther. (2010), https://doi.org/10.1089/hum.2010.173.
[21] A. Youssef, D. Aboalola, V.K.M. Han, The roles of insulin-like growth factors in mesenchymal stem cell niche, Stem Cells Int. (2017), https://doi.org/10.1155/ 2017/9453108.
[22] H.S. Kim, R.G. Rosenfeld, Y. Oh, Biological roles of insulin-like growth factor binding proteins (IGFBPs), Exp. Mol. Med. (1997), https://doi.org/10.1038/ emm.1997.13.
[23] E.J. Foulstone, P.B. Savage, A.L. Crown, J.M.P. Holly, C.E.H. Stewart, Role of insulin-like growth factor binding protein-3 (IGFBP-3) in the differentiation of primary human adult skeletal myoblasts, J. Cell. Physiol. 195 (2003) 70e79, https://doi.org/10.1002/jcp.10227.
[24] C. Aizawa, K. Saito, H. Ohshima, Regulation of IGF-I by IGFBP3 and IGFBP5 during odontoblast differentiation in mice, J. Oral Biosci. 61 (2019) 157e162, https://doi.org/10.1016/j.job.2019.07.001.
[25] K. Eguchi, Y. Akiba, N. Akiba, M. Nagasawa, L.F. Cooper, K. Uoshima, Insulin-like growth factor binding Protein-3 suppresses osteoblast differentiation via bone morphogenetic protein-2, Biochem. Biophys. Res. Commun. 507 (2018) 465e470, https://doi.org/10.1016/j.bbrc.2018.11.065.
[26] K. Hoa Nguyen, S. Mishra, B.L. Gregoire Nyomba, In vitro differentiation of mouse brown preadipocytes is enhanced by IGFBP-3 expression and reduced by IGFBP-3 silencing, Obesity 23 (2015) 2083e2092, https://doi.org/10.1002/ oby.21204.
[27] N. Sampson, C. Zenzmaier, M. Heitz, M. Hermann, E. Plas, G. Sch€afer, H. Klocker, P. Berger, Stromal insulin-like growth factor binding protein 3 (IGFBP3) is elevated in the diseased human prostate and promotes ex vivo fibroblast-to-myofibroblast differentiation, Endocrinology 154 (2013) 2586e2599, https://doi.org/10.1210/en.2012-2259.
[28] Y. Li, J. Xiang, C. Duan, Insulin-like growth factor-binding protein-3 plays an important role in regulating pharyngeal skeleton and inner ear formation and differentiation, J. Biol. Chem. 280 (2005) 3613e3620, https://doi.org/10.1074/ jbc.M411479200.
[29] L. Longobardi, L. O’Rear, S. Aakula, B. Johnstone, K. Shimer, A. Chytil, W.A. Horton, H.L. Moses, A. Spagnoli, Effect of IGF-I in the chondrogenesis of bone marrow mesenchymal stem cells in the presence or absence of TGF-b signaling, J. Bone Miner. Res. 21 (2006) 626e636, https://doi.org/10.1359/ jbmr.051213.
[30] A. Spagnoli, V. Hwa, W.A. Horton, G.P. Lunstrum, C.T. Roberts, F. Chiarelli, M. Torello, R.G. Rosenfeld, Antiproliferative effects of insulin-like growth factor-binding protein-3 in mesenchymal chondrogenic cell line RCJ3.1C5.18. Relationship to differentiation stage, J. Biol. Chem. (2001), https://doi.org/ 10.1074/jbc.M005088200.
[31] A. Spagnoli, M. Torello, S.R. Nagalla, W.A. Horton, P. Pattee, V. Hwa, F. Chiarelli, C.T. Roberts, R.G. Rosenfeld, Identification of STAT-1 as a molecular target of IGFBP-3 in the process of chondrogenesis, J. Biol. Chem. (2002), https:// doi.org/10.1074/jbc.M200218200.
[32] L. Longobardi, M. Torello, C. Buckway, L. O’Rear, W.A. Horton, V. Hwa, C.T. Roberts, F. Chiarelli, R.G. Rosenfeld, A. Spagnoli, A novel insulin-like growth factor (IGF)-independent role for IGF binding protein-3 in mesen-chymal chondroprogenitor cell apoptosis, Endocrinology 144 (2003) 1695e1702, https://doi.org/10.1210/en.2002-220959.
[33] A. Spagnoli, L. Longobardi, L. O’Rear, Cartilage disorders: potential therapeutic use of mesenchymal stem cells, in: IGF-I IGF Bind. Proteins, KARGER, Basel, 2005, pp. 17e30, https://doi.org/10.1159/000085719.
[34] L. O’Rear, L. Longobardi, M. Torello, B.K. Law, H.L. Moses, F. Chiarelli, A. Spagnoli, Signaling cross-talk between IGF-binding protein-3 and transforming growth factor-b in mesenchymal chondroprogenitor cell growth, J. Mol. Endocrinol. (2005), https://doi.org/10.1677/jme.1.01746.
[35] A.E. Grigoriadis, J.E. Aubin, J.N.M. Heersche, Effects of dexamethasone and vitamin D3 on cartilage differentiation in a clonal chondrogenic cell popula-tion, Endocrinology (1989), https://doi.org/10.1210/endo-125-4-2103.
[36] A.E. Grigoriadis, J.N.M. Heersche, J.E. Aubin, Analysis of chondroprogenitor frequency and cartilage differentiation in a novel family of clonal chondro-genic rat cell lines, Differentiation (1996), https://doi.org/10.1007/ s002580050160.
[37] T. Matsumoto, S.E. Gargosky, K. Iwasaki, R.G. Rosenfeld, Identification and characterization of insulin-like growth factors (IGFs), IGF-binding proteins (IGFBPs), and IGFBP proteases in human synovial fluid, J. Clin. Endocrinol. Metab. (1996), https://doi.org/10.1210/jc.81.1.150.
[38] S. Suzuki, S. Morimoto, M. Fujishiro, M. Kawasaki, K. Hayakawa, T. Miyashita, K. Ikeda, K. Miyazawa, M. Yanagida, K. Takamori, H. Ogawa, I. Sekigawa, Y. Takasaki, Inhibition of the insulin-like growth factor system is a potential therapy for rheumatoid arthritis, Autoimmunity (2015), https://doi.org/ 10.3109/08916934.2014.976631.
[39] T. Sato, K. Konomi, S. Yamasaki, S. Aratani, K. Tsuchimochi, M. Yokouchi, K. Masuko-Hongo, N. Yagishita, H. Nakamura, S. Komiya, M. Beppu, H. Aoki, K. Nishioka, T. Nakajima, Comparative analysis of gene expression profiles in intact and damaged regions of human osteoarthritic cartilage, Arthritis Rheum. (2006), https://doi.org/10.1002/art.21638.
[40] E.B. Hunziker, E. Kapfinger, J. Martin, J. Buckwalter, T.I. Morales, Insulin-like growth factor (IGF)-binding protein-3 (IGFBP-3) is closely associated with the chondrocyte nucleus in human articular cartilage, Osteoarthr. Cartil. 16 (2008) 185e194, https://doi.org/10.1016/j.joca.2007.06.008.
[41] Z. Wei, H.H. Li, IGFBP-3 may trigger osteoarthritis by inducing apoptosis of chondrocytes through Nur77 translocation, Int. J. Clin. Exp. Pathol. (2015).
[42] D. Seol, D.J. McCabe, H. Choe, H. Zheng, Y. Yu, K. Jang, M.W. Walter, A.D. Lehman, L. Ding, J.A. Buckwalter, J.A. Martin, Chondrogenic progenitor cells respond to cartilage injury, Arthritis Rheum. (2012), https://doi.org/ 10.1002/art.34613.
[43] D.S. Evans, F. Cailotto, N. Parimi, A.M. Valdes, M.C. Castano~-Betancourt, Y. Liu, R.C. Kaplan, M. Bidlingmaier, R.S. Vasan, A. Teumer, G.J. Tranah, M.C. Nevitt, S.R. Cummings, E.S. Orwoll, E. Barrett-Connor, J.B. Renner, J.M. Jordan, M. Doherty, S.A. Doherty, A.G. Uitterlinden, J.B.J. Van Meurs, T.D. Spector, R.J. Lories, N.E. Lane, Genome-wide association and functional studies identify a role for IGFBP3 in hip osteoarthritis, Ann. Rheum. Dis. (2015), https:// doi.org/10.1136/annrheumdis-2013-205020.
[44] L. Ho, A. Stojanovski, H. Whetstone, Q.X. Wei, E. Mau, J.S. Wunder, B. Alman, Gli2 and P53 cooperate to regulate IGFBP-3- mediated chondrocyte apoptosis in the progression from benign to malignant cartilage tumors, Canc. Cell (2009), https://doi.org/10.1016/j.ccr.2009.05.013.